|
Human Genome Data in Relation to Disease Management and Control
Prof. Bharathi P.Salimath
Department of Applied Botany and Biotechnology,
University of Mysore,
Manasagangotri,
Mysore-570006.
Cells are the fundamental working units of every living system. All the instructions needed to direct their activities are contained within deoxyribonucleic acid (DNA). A genome is all the DNA in an organism, including its genes. Genes carry information for making proteins required by all organisms. It is the proteins that perform most life functions and even make up the majority of cellular structures. DNA is made up of four bases A, T, C & G that are repeated millions of billions of times throughout a genome. The human genome has 3 billion pairs of bases. The particular order of As, Ts, Cs and Gs is extremely important. The order underlies all of life's diversity, even dictating whether an organism is human or another species such as yeast, rice or fruit fly.
The human genome project (HGP) is one of the great feats of exploration in history. HGP is coordinated by the U.S. Department of Energy and the National Institutes of Health that officially began in 1990.
The goals of the HGP are to:
* Identify all the approximate 30,000 genes in human DNA.
* Determine the sequences of the 3 billion chemical base pairs that make up human DNA.
* Store this data in databases.
* Improve tools for data analysis.
* Transfer related technologies to the private sector, &
* Address the ethical, legal and social issues (ELS1) that may arise from the project.
* To sequence genomes of model organisms (E.coli, fruit fly and mouse) to help interpret human DNA.
In February 2001, HGP and Celera Genomics scientists published the long-awaited details of the working-draft DNA sequence. An international research effort to sequence and map all of the genes together known as the genome-of the members of our species. Homosapiens will be finished to high quality by 2003. Once complete, it will give us the chance for the first time to read natures complete genetic blueprint for building a human being.
The following methodologies are used to sequence a genome
* Mapping
* Building libraries
* Sub-cloning
* Storing and copying DNA in E.coli
* Preparing DNA for sequencing
* Sequencing reaction and product production
* Separating the sequencing reaction
* Reading the sequencing reaction
* Assembling the results
* Working draft sequence/ conclusion.
The working draft tells us the following:
* The human genome contains 3164.7 million chemical nucleotide bases (A, C, T, and G).
* The average gene consists of 3000 bases, but sizes vary greatly, with the largest known human
gene being dystrophin at 2.4 million bases.
* The total number of genes is estimated at 30,000 to 35,000 much lower than previous estimates of
80,000 to 140,000 that had been based on extrapolations from gene-rich areas as opposed to a
composite of gene-rich and gene-poor areas.
* Almost all (99.9%) nucleotide bases are exactly the same in all people.
* The functions are unknown for over 50% of discovered genes.
* Less than 2% of the genome codes for proteins.
* Repeated sequences that do not code for proteins ("junk DNA") make up at least 50% of the human
genome.
* Repetitive sequences are thought to have no direct functions, but they shed light on chromosome
structure and dynamics. Over time, these repeats reshape the genome by rearranging it, creating
entirely new genes, and modifying and reshuffling existing genes.
* During the past 50 million years, a dramatic decrease seems to have occurred in the rate of
accumulation of repeats in the human genome.
* The human genome's gene-dense "urban centers" are predominantly composed of the DNA
building blocks G and C.
* In contrast, the gene-poor "deserts" are rich in the DNA building blocks A and T. GC- and AT-rich
regions usually can be seen through a microscope as light and dark bands on chromosomes.
* Genes appear to be concentrated in random areas along the genome, with vast expanses of
noncoding DNA between.
* Stretches of up to 30,000 C and G bases repeating over and over often occur adjacent to gene-rich
areas, forming a barrier between the genes and the "junk DNA." These CpG islands are believed to
help regulate gene activity.
* Chromosome 1 has the most genes (2968), and the Y chromosome has the fewest (231).
How the Human Compares with Other Organisms
* Unlike the human's seemingly random distribution of gene-rich areas, many other organisms'
genomes are more uniform, with genes evenly spaced throughout.
* Humans have on average three times as many kinds of proteins as the fly or worm because of
mRNA transcript "alternative splicing" and chemical modifications to the proteins. This process can
yield different protein products from the same gene.
* Humans share most of the same protein families with worms, flies, and plants, but the number of
gene family members has expanded in humans, especially in proteins involved in development and
immunity.
* The human genome has a much greater portion (50%) of repeat sequences than the mustard weed
(11%), the worm (7%), and the fly (3%).
* Although humans appear to have stopped accumulating repeated DNA over 50 million years ago,
there seems to be no such decline in rodents. This may account for some of the fundamental
differences between hominids and rodents, although gene estimates are similar in these species.
Scientists have proposed many theories to explain evolutionary contrasts between humans and
other organisms, including those of life span, litter sizes, inbreeding, and genetic drift.
Variations and Mutations
* Scientists have identified about 1.4 million locations where single-base DNA differences (SNPs)
occur in humans. This information promises to revolutionize the processes of finding chromosomal
locations for disease-associated sequences and tracing human history.
* The ratio of germline (sperm or egg cell) mutations is 2:1 in males vs females. Researchers point to
several reasons for the higher mutation rate in the male germline, including the greater number of
cell divisions required for sperm formation than for eggs.
Will understanding the human genome transform preventive, diagnostic and therapeutic medicine?
Diagnosing and Predicting Disease and Disease Susceptibility
All diseases have a genetic component, whether inherited or resulting from the body's response to environmental stresses like viruses or toxins. The successes of the Human Genome Project (HGP) have even enabled researchers to pinpoint errors in genes--the smallest units of heredity--that cause or contribute to disease.
The ultimate goal is to use this information to develop new ways to treat, cure, or even prevent the thousands of diseases that afflict humankind. But the road from gene identification to effective treatments is long and fraught with challenges. In the meantime, biotechnology companies are racing ahead with commercialization by designing diagnostic tests to detect errant genes in people suspected of having particular diseases or at risk for developing them.
An increasing number of gene tests are becoming available commercially although the scientific community continues to debate the best way to deliver them to the public and medical communities that are often unaware of their scientific and social implications. While some of these tests have greatly improved and even saved lives, scientists remain unsure of how to interpret many of them. Also, patients taking the tests face significant risks of jeopardizing their employment or insurance status. And because genetic information is shared, these risks can extend beyond them to their family members as well.
Disease Intervention
Within the next decade, researchers will find most human genes. Explorations into the function of each one --a major challenge extending far into the 21st century --will shed light on how faulty genes play a role in disease causation. With this knowledge, commercial efforts will shift away from diagnostics and toward developing a new generation of therapeutics based on genes. Drug design will be revolutionized as researchers create new classes of medicines based on a reasoned approach using gene sequence and protein structure function information rather than the traditional trial-and-error method. The drugs, targeted to specific sites in the body, promise to have fewer side effects than many of today's medicines.
The potential for using genes themselves to treat disease--known as gene therapy--is the most exciting application of DNA science. It has captured the imaginations of the public and the biomedical community for good reason. This rapidly developing field holds great potential for treating or even curing genetic and acquired diseases, using normal genes to replace or supplement a defective gene or to bolster immunity to disease (e.g., by adding a gene that suppresses tumor growth).
One of the greatest impacts of having the sequence may well be in enabling an entirely new approach to biological research. Within 20 years, novel drugs will be available that derive from a detailed molecular understanding of common illnesses like diabetes and high blood pressure. The drugs will be designer therapies that target molecules logically and are therefore potent without significant side effects. Drugs like those for cancer will routinely be matched to a patient's likely response, as predicted by molecular fingerprinting. Diagnoses of many conditions will be much more thorough and specific than now. For example, a patient who learns that he has high cholesterol will also know which genes are responsible, what effect the high cholesterol is likely to have, and what diet and pharmacologic measures will work best for him.
By 2050, many potential diseases will be cured at the molecular level before they arise, though large inequities worldwide in access to these advances will continue to stir tensions. When people become sick, gene therapies and drug therapies will home in on individual genes, as they exist in individual people, making for precise, customized medical treatment. The average life span will reach 90 to 95 years, and a detailed understanding of human aging genes will spur efforts to expand the maximum span of human life.
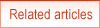
|
|