|
Bioreactors
Prof. Jayant M. Modak
Department of Chemical Engineering
Indian Institute of Science,
Bangalore 560012
Email: modak@chemeng.iisc.ernet.in
1 Introduction
Biotechnology is the culmination of more than 8000 years of human experience using living organisms and the process of fermentation to make products such as bread, cheese, beer and wine. Today biotechnology is applied to manufacturing processes used in health care, food and agriculture, industrial and environmental cleanup, among other applications. The word biotechnology, contraction of biological technology, came in general use in mid 1970's. Over the year’s at least 10 different definitions of biotechnology has been proposed. A widely accepted definition of Biotechnology is "Application of scientific and engineering principles to processing of materials by biological agents to provide goods and service". Some other definitions replace rather ambiguous word ‘biological agents’ with more specific words such as microorganisms, cells, plant and animal cells and enzymes. Biotechnology is truly an interdisciplinary field with contributions from basic life science disciplines such as molecular and cell biology, biochemistry, genetics and engineering such as chemical, instrumentation and control. In this section, a brief summary of biotechnological applications, modeling preliminaries and different types of bioreactors is presented. When a biotechnological process is implemented on a commercial scale there is every reason to believe that it will the in some bioreactor or fermenter. The entire process can be divided in three stages.
Stage I : Upstream processing which involves preparation of liquid medium, separation of particulate and inhibitory chemicals from the medium, sterilization, air purification etc.,
Stage II: Fermentation which involves the conversion of substrates to desired product with the help of biological agents such as microorganisms; and
Stage III: Downstream processing which involves separation of cells from the fermentation broth, purification and concentration of desired product and waste disposal or recycle.
Depending on the type of product, the concentration levels it is produced and the purity desired, the fermentation stage might constitute anywhere between 5-50% of the total fixed and operating costs of the process. Therefore, optimal design and operation of bioreactor frequently dominates the overall technological and economic performance of the process.
2. Bioreactors
At every step of the development of a biotechnological process, bioreactor is invariably used. The sizes of the bioreactor can vary over several orders of magnitudes. The microbial cell (few mm3), shake flask ( 100-1000 ml), laboratory fermenter ( 1 – 50 L), pilot scale (0.3 – 10 m3) to plant scale ( 2 – 500 m3) are all examples of bioreactors. Whatever may be the size of the bioreactor, the conditions in the bioreactor have to be favorable so that living microorganisms can exhibit their activity (specific biochemical and microbial reactions) under defined conditions. This results in a series of special features in the reaction engineering of biocatalytic processes. The reaction rate, cell growth, and process stability depend on the environmental conditions in the bioreactor. There are several unique aspects of biotechnological processes, which require special consideration in design of bioreactors.
2.1 Unique aspects of biological processes
(a) The concentrations of starting materials (substrates) and products in the reaction mixture are frequently low; both the substrates and the products may inhibit the process. Cell growth, the structure of intracellular enzymes, and product formation depend on the nutritional needs of the cell (salts, oxygen) and on the maintenance of optimum biological conditions (temperature, concentration of reactants, and pH) with in narrow limits.
(b) Certain substances inhibitors effectors, precursors, metabolic products influence the rate and the mechanism of the reactions and intracellular regulation.
(c) Microorganisms can metabolize unconventional or even contaminated raw materials (cellulose, molasses, mineral oil, starch, ores, wastewater, exhaust air, biogenic waste), a process which is frequently carried out in highly viscous, non-Newtonian media.
(d) In contrast to isolated enzymes or chemical catalysts, microorganisms adapt the structure and activity of their enzymes to the process conditions, whereby selectivity and productivity can change. Mutations of the microorganisms can occur under sub optimal biological conditions.
(e) Microorganisms are frequently sensitive to strong shear stress and to thermal and chemical influences.
(f) Reactions generally occur in gas-liquid -solid systems, the liquid phase usually being aqueous.
(g) The microbial mass can increase as biochemical conversion progresses. Effects such as growth on the walls, flocculation, or autolysis of microorganisms can occur during the reaction.
(h) Continuous bioreactors often exhibit complicated dynamic behavior.
2.2 Requirements of bioreactors
Due to above mentioned demands made by biological systems on their environment, there is no universal bioreactor. However, the general requirements of the bioreactor are as follows:
(a) The design and construction of biochemical reactors must preclude foreign contamination (sterility). Furthermore, monoseptic conditions should be maintained during the fermentation and ensure containment.
(b) Optimal mixing with low, uniform shear
(c) Adequate mass transfer (oxygen)
(d) Clearly defined flow conditions
(e) Feeding of substrate with prevention of under or overdosing
(f) Suspension of solids
(g) Gentle heat transfer
(h) Compliance with design requirements such as: ability to be sterilized; simple construction; simple measuring, control, regulating techniques; scaleup; flexibility; long term stability; compatibility with up- downstream processes; antifoaming measures.
2.3 Types of bioreactors
Bioreactors can be classified according to various different criteria
(a) Type and form of biocatalyst: free cells in submerged cultures; carried bound or immobilized cells/enzymes; retention or recirculation of the biocatalyst
(b) Configuration: tank (height/diameter <3), column (height/diameter > 3)
(c) Energy input and aeration: liquid phase; gas phase; combined
(d) Hydrodynamics: perfect mixing; partial mixing; no mixing;
(e) Mode of operation: batch; continuous; fed-batch.
Few of the bioreactor types are discussed below:
Stirred tank Bioreactors (STB)
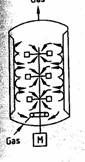
Microbial fermentations received prominence during 1940's namely for the production of life saving antibiotics. Stirred tank reactor is the choice for many (more than 70%) though it is not the best. STB’s have the following functions: homogenization, suspension of solids, dispersion of gas-liquid mixtures, aeration of liquid and heat exchange. The STB is provided with a baffle and a rotating stirrer is attached either at the top or at the bottom of the bioreactor. The typical decision variables are: type, size, location and the number of impellers; sparger size and location. These determine the hydrodynamic pattern in the reactor, which in turn influence mixing times, mass and heat transfer coefficients, shear rates etc. The conventional fermentation is carried out in a batch mode. Since stirred tank reactors are commonly used for batch processes with slight modifications, these reactors are simple in design and easier to operate. Many of the industrial bioprocesses even today are being carried out in batch reactors though significant developments have taken place in the recent years in reactor design, the industry, still prefers stirred tanks because in case of contamination or any other substandard product formation the loss is minimal. The batch stirred tanks generally suffer due to their low volumetric productivity. The downtimes are quite large and unsteady state fermentation imposes stress to the microbial cultures due to nutritional limitations. The fed batch mode adopted in the recent years eliminates this limitation. The STBs offer excellent mixing and reasonably good mass transfer rates. The cost of operation is lower and the reactors can be used with a variety of microbial species. Since stirred tank reactor is commonly used in chemical industry the mixing concepts are well developed. STR with immobilized cells is not favored generally due to attrition problems, however by separating the zone of mixing from the zone of cell culturing one can successfully operate the system.
Airlift bioreactors (ALB)
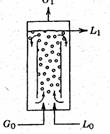
Airlift bioreactors (ALB) are generally classified as pneumatic reactors without any mechanical stirring arrangements for mixing. The turbulence caused by the fluid flow ensures adequate mixing of the liquid. The draft tube is provided in the central section of the reactor. The introduction of the fluid (air/liquid) causes upward motion and results in circulatory flow in the entire reactor. The air/liquid velocities will be low and hence the energy consumption is also low. ALBs can be used for both free and immobilized cells. There are very few reports on ALBs for metabolite production. The advantages of Airlift reactors are the elimination of attrition effects generally encountered in mechanical agitated reactors. It is ideally suited for aerobic cultures since oxygen mass transfer coefficient are quite high in comparison to stirred tank reactors.
Fluidized bed Bioreactors (FBB)
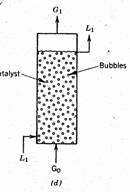
Fluidized bed bioreactors (FBB) have received increased attention in the recent years due to their advantages over other types of reactors. Most of the FBBs developed for biological systems involving cells as biocatalysts are three phase systems (solid, liquid & gas). The fundamentals of three phase fluidization phenomena have been comprehensively covered in chemical engineering literature. The FBBs are generally operated in co-current upflow with liquid as continuous phase and other more unusual configurations like the inverse three phase fluidized bed or gas solid fluidized bed are not of much importance. Usually fluidization is obtained either by external liquid re-circulation or by gas fed to the reactor. In the case of immobilized enzymes the usual situation is of two-phase systems involving solid and liquid but the use of aerobic biocatalyst necessitate introduction of gas (air) as the third phase. A differentiation between the three phase fluidized bed and the airlift bioreactor would be made on the basis that the latter have a physical internal arrangement (draft tube), which provides aerating and non-aerating zones. The circulatory motion of the liquid is induced due to the draft tube. Basically the particles used in FBBs can be of three different types: (i) inert core on which the biomass is created by cell attachment. (ii) Porous particles in which the biocatalyst is entrapped.(iii) Cell aggregates/ flocs (self-immobilization). In comparison to conventional mechanically stirred reactors, FBBs provide a much lower attrition of solid particles. The biocatalyst concentration can significantly be higher and washout limitations of free cell systems can be overcome. In comparison to packed bed reactors FBBs can be operated with smaller size particles without the drawbacks of clogging, high liquid pressure drop, channeling and bed compaction. The smaller particle size facilitates higher mass transfer rates and better mixing. The volumetric productivity attained in FBBs is usually higher than in stirred tank and packed bed bioreactors. There are several successful examples of using FBBs in bioprocess development.
Packed bed bioreactors
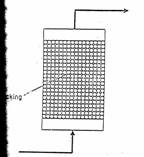
Packed bed or fixed bed bioreactors are commonly used with attached biofilms especially in wastewater engineering. The use of packed bed reactors gained importance after the potential of whole cell immobilization technique has been demonstrated. The
immobilized biocatalyst is packed in the column and fed with nutrients either from top or from bottom. One of the disadvantages of packed beds is the changed flow characteristic due to alterations in the bed porosity during operation. While working with soft gels like alginates, carragenan etc the bed compaction which generally occurs during fermentation results in high pressure drop across the bed. In many cases the bed compaction was so severe that the gel integrity was severely hampered. In addition channeling may occur due to turbulence in the bed. Though packed beds belong to the class of plug flow reactors in which backmixing is absent in many of the packed beds slight amount of backmixing occurs which changes the characteristics of fermentation. Packed beds arc generally used where substrate inhibition governs the rate of reaction. The packed bed reactors are widely used with immobilized cells. Several modifications such as tapered beds to reduce the pressure drop across the length of the reactor, inclined bed, horizontal bed, rotary horizontal reactors have been tried with limited success.
3. Selection of Bioreactor
The preliminary choice of bioreactor depends on the number of factors some of which are listed below:
Reactor and processing
Area or application
(research, production) |
reactor dimensions, material, mode of operation, instrumentation |
Value or desired product |
material, size, mode or operation, instrumentation |
Processing
(batch, fed- batch, continuous) |
reactor dimensions, combination or reactors, instrumentation |
Subsequent downstream
processing |
mode or operation, auxiliary devices, special designs, membrane reactors |
Product formation kinetics |
mode or operation, reactor type, reactor combination, flow patterns |
Culture and culture medium
Metabolic state
(aerobic, microaerobic, anaerobic) |
oxygen input, reactor type, seals
|
Physiological state (viable,
dead, growing, resting) |
cell retention, oxygen input |
Morphology (spherical,
filamentous, flocs, films) |
dispersing device, energy input, reactor geometry, reactor type |
Sterility (sterile, nonsterile) |
materials, fittings, seals, sterile techniques, sampling, auxiliary devices |
Properties
Physicochemical (gaseous,
liquid, solid, multiphase) |
dispersing device, internals, cooling, processing |
Biological (inhibition,
mechanical stress) |
substrate dosage, energy dissipation |
Rheological (high, low viscosity) |
dispersing device, reactor type and geometry, internals |
Bubbles (promoting or
inhibiting coalescence) |
shape and design or gassing device, foam control, bubble dispersion |
Foam formation |
gas sparger, reactor design, flow patterns, antifoaming agent |
General References
Bailey J.E. and Ollis D.F., Biochemical Engineering Fundamentals, McGraw Hill 1986.
Shuler, M. L. and F. Kargi, Bioprocess Engineering, 1992
Rehm, H. J and Reed, G., Biotechnology - A Comprehensive Treatise, 1982.
Perry and Chilton, Chemical Engineering Handbook, 6th edition
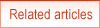
|
|