|
NMR Spectroscopy and Biomolecular Structure
N. Suryaprakash,
Sophisticated Instruments Facility,
Indian Institute of Science,
Bangalore 560 012,
11th December 2002 )
Till late 1980’s, the only method that was available to determine the structure of biomolecules like proteins was X-ray crystallography. In spite of the fact that one of the prerequisite for the determination of the structure is the availability of single crystals, this method is by and large adopted as the major technique.
The development of multidimensional Nuclear Magnetic Resonance (NMR) spectroscopy in recent years resulted in exploiting this technique for the determination of three dimensional structures of proteins. In fact the Nobel prize for the year 2002 in chemistry is given to Prof. Kurt Wuthrich for his work on “Three dimensional Structure of Biomolecules”.
The biggest advantage of using NMR spectroscopy is that one can obtain the 3D structure in water, which is the solvent in which most biological reactions take place (enzymes and drugs interact in water). Moreover it gives the dynamic information of the molecule. It is not that NMR spectroscopy can give all the information. It has its own limitations. As the protein size becomes bigger and bigger, the resonance assignments are not very easy, although part of the problems are overcome in recent years by tremendous development in experimental schemes. Recent published work is on a protein of 81.4 kD having nearly 723 residues (J. Am. Chem. Soc. 124, 10023-35 (2002).
Introduction to NMR Spectroscopy
The NMR spectroscopy started with a Nobel Prize winning discovery by physicists in 1946 was encroached by chemists and is being exploited by biologists. The basic requirement for NMR study is that nucleus should possess spin quantum number (I). (1) Nuclei with Even atomic mass & number Eg. (12C, 16O) have their spin zero (I = 0), (2) Nuclei with even atomic mass & odd number (14N, 2H, 10B) have their spin as integer multiples and (3) nuclei with odd atomic mass and even or odd number (1H, 13C, 15N, 31P) have half integer spins. The nuclei belong to category 2 and 3 are called NMR active. Each nuclear spin can be treated like a tiny magnet. Once we say they are magnets, then we have to talk about magnetic moment. Just like the spin is an intrinsic property of a fundamental particle, magnetic moment is also an inherent property of spins. In a given molecule, in the absence of the magnetic field, the spins are oriented in all the directions and there is no energy difference between them. However, when placed in a magnetic field, they always try to orient along or opposite to the direction of the magnetic field. Generally, more spins are aligned in the direction of the magnetic field than opposing them. This creates an energy difference between the orientations. Since energy is related to the frequency, we can write the energy separation in terms of frequency as,
DE = h n
n = g Bo / 2p
The frequency n is proportional to the magnetic field and the proportionality constant is called the gyromagnetic ratio (g). This gyromagnetic ratio varies with the nuclei.
Substituting this in the above equation, we get,
DE = g h Bo / 2p
That means energy separation varies with the nuclei in a given magnetic field. All I am trying to say now is that one can study all the NMR nuclei of a molecule.
Next step is to detect the NMR signal. The energy separation when expressed in frequency, we find it is in the radio frequency range of the electromagnetic spectrum. If we now apply an external radio frequency (rf) in a direction perpendicular to the magnetic field (right hand thumb rule applies), say X-axis, we can tilt the nuclear spins, also called bulk magnetization from Z-axis to the direction of Y-axis. If we place our detector along this axis, we can detect the NMR signal. If the frequency of incident rf, matches with the energy separation, there will be absorption of energy by the spins, which finally result in inducing emf in the detector. Since it is to do with the magnetic moment of the nucleus and we see resonance, it is called Nuclear Magnetic Resonance. Let us not go more into the details of NMR as it is a field by itself.
What we should know is how we can exploit the technique to determine the structure of molecules. In this context, we should remember couple of terms, which are different types of interaction of the nuclei in a magnetic field. Let us restrict to only two of them.
(1) Chemical Shift : This is to do with the interaction of the nuclear spins with the electronic field surrounding it. This is what makes NMR technique very powerful. Different chemical groups in the molecule have characteristic chemical shifts and making the resonance assignment to these chemical groups, we can determine the structure.
Spin-Spin Coupling : This is the interaction of one nuclear spin with the other through chemical bond. Coupling patterns are crucial to identify spin systems in a molecule and for the determination of its chemical structure
That is just a bit of NMR. Let us see how we can use it in the biomolecular structure determination.
Structures of biomolecules
Let us take the example of a protein structure. If we understand the steps involved, then basically the technique can be employed for the structure determination, wherever feasible. What are the things we need to know in protein structure?
Primary structure : It is nothing but the sequence of amino acids in the chain. It always starts from N terminal (NH2) to C terminal (CO group)
Secondary structure : It tell us the way the amino acid chains are arranged locally. There are several examples, like a -helix, b-sheet, b-turn, etc.
Tertiary structure : Tells how all the elements of the secondary structure come together in solution. In short, how the whole thing packs (or not) in solution, eg. whether the protein folds or not.
We will keep aside the quaternary structure for now.
How we can use NMR to determine these structures? What is the procedure?
Prerequisite for the structural study is the amino acid sequence. That is the knowledge of primary structure.
All the amino acid residues in the peptide are separated by the peptide bond. In other words in NMR terminology, we say the spin systems for the different residues are isolated from one another. With the knowledge of the chemical shifts of different protons in amino acids like, Ca,Cb, Cg, Cd, NH and other aromatic groups, one can make assignment of resonances. If the molecule is very small, say 4-5 residue, it is straightforward. When the number of residue increases, complete assignment using one dimensional proton spectra is very difficult. Then we resort to sophisticated experiments using “Two dimensional NMR”.
Two dimensional NMR
In conventional NMR, the resonance frequencies (chemical shifts) are plotted as a function of their intensities. In two dimensional NMR, the intensities are plotted as a function of two frequencies. There are several ways of doing this. In simple terms the basic two dimensional experiment is given in four time periods as
Preparatory period evolution period mixing period detection period
t1 t2
In the preparation period the sample is excited by one or more pulses. During the time period t1 the sample is allowed to evolve. Mixing period consists of one or more pulses depending the information we are seeking. Finally the magnetization is detected during the detection period. Depending on what is done in the preparation and mixing period, we can design experiments to derive the information of interest. The two dimensional Fourier transform of the data collected during t2 as a function of t1 give a 2D spectrum where the information is spread in two dimensions. The point to be remembered is the data is collected only in t2 and not in t1 but as a function of t1.
There are several two dimensional experiments designed depending on the choice of preparation and mixing periods. Some of them which are of most important for the structural studies in biomolcules are
COSY : Abbreviation for COrrelated SpectroscopY
This is the simplest of the 2D experiments consists of only two pulses with an incremental delay (t1) between them. Provides information on the spins coupled to each other. The first pulse creates magnetization in the transverse plane. During the evolution period the delay is incremented systematically to sample the spectra in the other dimension also. The second pulse mixes the magnetization between the spins coupled to each other. The double Fourier transform of this gives a spectra, where the diagonal looks alike with the normal one dimensional spectrum. The off-diagonal peaks (called cross peaks) gives information on the nuclei which were coupled to each other through chemical bond.
TOCSY : Abbreviation for TOtal Correlated SpectroscopY
In this experiment, there will be a mixing period after the evolution period. During the mixing time the magnetization exchange through scalar coupling. Depending on the length of the mixing time, the magnetization is transferred between all the coupled nuclei in a spin system, even if they are not directly coupled. This essentially gives the same information as that of COSY, except that COSY gives information only on the directly coupled spins, whereas TOCSY gives the complete spin coupling network.
NOESY : Abbreviation for Nuclear Overhauser Effect SpectroscopY
NOESY is one of the most useful experiment as it is used to correlate the nuclei through space. This gives qualitative and quantitative information on the proximity of two nuclei. Also by measuring the intensity of the peaks, the distance information can be obtained. In this experiment after magnetization evolves during t1 period, exchange of the magnetization takes place during the mixing time through a dipole-dipole relaxation mediated relaxation.
Structure determination
All structural information is available once firm assignments of the residues are made using 2D technique. This is the crucial step. It is necessary not only to identify every amino acid type but also by its position in the polypeptide sequence. The chemical shift positions of Ca proton chemical shifts are very useful and secondary structure has characteristic pattern in this region. a helices have little chemical shift dispersion whereas b sheet have more dispersion. Second important step is to NOE parameters. It is most useful for tertiary structure determination.
Typical flow chart followed for tertiary structure determination
1. Sequence specific assignments
Make use of experiments like COSY, TOCSY, DQFC, etc..
2. Collect restraints from NOE
Make use of experiments like NOESY, ROESY, etc…
Generally other constraints are also used like Hydrogen bond, Torsion constraints, etc. (I am not going into details of all these due to limited time)
3. Secondary structure determination
Generally characteristic patterns exist for secondary structure in finger pring region
4. The constraints obtained are used in certain distance geometry algorithm to obtain tertiary structure
5. Refinement of the structure using molecular dynamics / molecular modelling
The procedure for the determination of structure of biomolecule is same whether it is polypeptide or protein. So far what I said is the preliminary steps. In obtaining the real structure, one has to carry out several more experiments designed to retrieve information. I am not covering all of them due to lack of time. Depending on the system one can choose several other sophisticated experiments involving multi dimensions in structure determination.
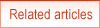
|
|